Friday, March 31, 2006
The best of recent science-related blogging
Check out the latest editions of science-related blog carnivals:
Tangled Bank (science, nature, medicine and environment)
Grand Rounds (medicine and healthcare)
Carnival of the Green (environment and conservation)
Circus of the Spineless (invertebrates)
I And The Bird (birds and birdwatching)
Animalcules (microorganisms)
Skeptic's Circle (dissecting pseudoscience and medical quackery)
Carnival of Education (education)
Tangled Bank (science, nature, medicine and environment)
Grand Rounds (medicine and healthcare)
Carnival of the Green (environment and conservation)
Circus of the Spineless (invertebrates)
I And The Bird (birds and birdwatching)
Animalcules (microorganisms)
Skeptic's Circle (dissecting pseudoscience and medical quackery)
Carnival of Education (education)
Thursday, March 30, 2006
Do sponges have circadian clocks?
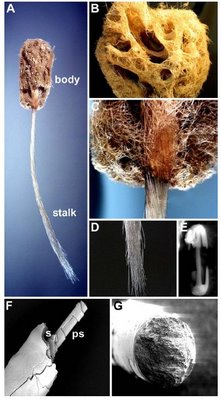
Should one expect sponges to have circadian clock? Considering that every animal, plant, fungus and protist, as well as some bacteria have clocks, it would be more surprising if sponges did not have one.
I've been on a lookout, for quite some time now, for any mention in the literature of a possible daily rhythm in sponges. There is not much, but there is some. What is known for sure, is that both adult [1] and larval [2] sponges perceive light, thus they should be capable of entraining to the environmental light-dark cycles.
In the field, sponges release their larvae (spawn) in a manner that suggests that they a) can percieve environmetal light, b) use such information to calculate the time of day, and c) also have a lunar rhythm (either an endogenous circalunar clock entrained by the moon, or exogenous rhythm driven directly by moonlight):
Release started every day of 3 3-d period (12 to 14 October) at about 1400 hrs and lasted until just after sunset (1830 hrs). Ninety percent of the population showed reproductive activity. Exactly one lunar month later (11 to 12 November), a second release of gametes occurred. In the following year the same sequence of events was observed for the original population (2 to 4 October and 1 to 2 November, 1985). In all instances the first gamete release began on the third day after the full moon. These and earlier observations on this phenomenon show a strong correlation between moon phase and the time of gamete release [3].Another interesting behavior in sponges is called "pumping". Those are the contractions of the whole body of the sponge. Apparently [4], the contractions are slower during the night than during the day, indicating a diurnal, if not a circadian rhythm. Here is a pattern recorded from one sponge in the lab:
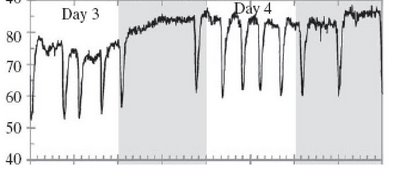
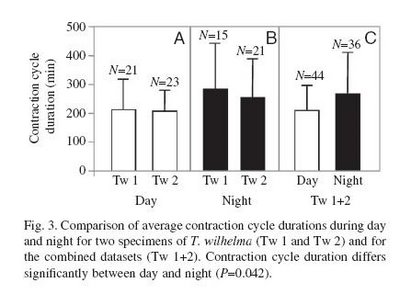
If you did not know this before, sponges can move. Not fast enough to grab your ankle while swimming - more on the order of 6mm per day. This was a serendipitous finding a couple of decades ago, about a mile from where I live now, at UNC-Chapel Hill.
Calhoun Bond, then doing his PhD here (and now teaching at Greensboro College), happened to have an aquarium in his office. One day he looked at it and noticed that a sponge was not in the same place where it was before. So, he got a multi-million-dollar grant needed to buy a Sharpie pen [ ;-) ] and started drawing the position of the sponge on the glass wall of the aquarium. Yup, the sponge sure moved:

Dr.Bond has since published a couple of papers on the exact mechanim the sponge moves. It does not have a moving "leg" on the bottom. Instead, the whole animal rearranges itself as cells move over each other, pulling the spikules along. I've seen the stop-motion movies and they are impressive.
He has not noticed, though, if the sponge had different speeds during the day as opposed to during the night, but this could be easily done by an undegraduate student in a biology lab. Instead of drawing a mark once per day, draw a mark every 3-4 hours over a period of a couple of days, and the answer will be there. If there is a day/night difference in the sponge locomotion speed in a light-dark cycle, then it would be worth running the sponges through a standard battery of tests, including phase-shifts of the light-dark cycle, exposure to constant dark and constant light, and phase-shifting the rhythm with light pulses. This process could even be automated with a camera which can allow one to monitor the behavior over longer periods of time (e.g,. weeks or months).
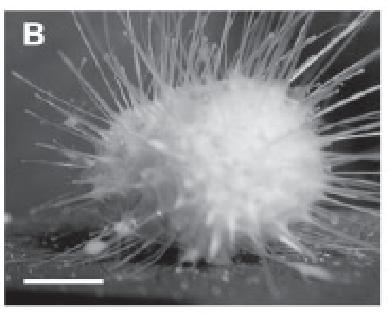
One thing that I am surprised nobody did so far was to see if sponges have core circadian clock genes, like period, timeless, clock and cryptochrome. It is apprently relatively easy technically to do so. I have seen phylogenetic analyses of sequences of clock genes in dozens of, for instance, insect species. It can't be that hard to take a look at a sponge and, if teh clock genes are there, see in which cells and with what temporal dynamics they are expressed.
[1] Werner E.G. Muller, Klaus Wendt, Christopher Geppert, Matthias Wiens, Andreas Reiber, Heinz C. Schroder, Novel photoreception system in sponges? Unique transmission properties of the stalk spicules from the hexactinellid Hyalonema sieboldi, Biosensors and Bioelectronics 21 (2006) 1149–1155
[2] Sally P. Leys and Bernard M. Degnan, Cytological Basis of Photoresponsive Behavior in a Sponge Larva, Biol. Bull. 201: 323-338. (December 2001)
[3] W. F. Hoppe and M. J. M. Reichert, Predictable annual mass release of gametes by the coral reef sponge Neofibularia nolitangere (Porifera: Demospongiae), Marine Biology, Volume 94, Number 2, 277 - 285 (March 1987)
[4] Michael Nickel, Kinetics and rhythm of body contractions in the sponge Tethya wilhelma
(Porifera: Demospongiae), The Journal of Experimental Biology 207, 4515-4524
[5] CALHOUN BOND AND ALBERT K. HARRIS, Locomotion of Sponges and Its Physical Mechanism, THE JOURNAL OF EXPERIMENTAL ZOOLOGY 246:271-284 (1988)
[6] CALHOUN BOND, Continuous Cell Movements Rearrange Anatomical - Structures in Intact Sponges, THE JOURNAL OF EXPERIMENTAL ZOOLOGY 263:284-302 (1992)
[7] Amano, S, Morning release of larvae controlled by the light in an intertidal sponge, Callyspongia ramosa . Biological Bulletin, Marine Biological Laboratory, Woods Hole. Vol. 175, pp. 181-184. (1988).
Tuesday, March 28, 2006
National Sleep Awareness Week
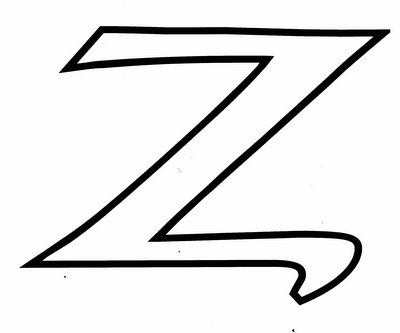
This week (March 27, 2006 - April 2, 2006) is the National Sleep Awareness Week. So, I assume that Circadiana will get a lot of hits this week. Perhaps if you all link to it....(hint, hint LOL)
ClockNews - Adolescent Sleep
Health Journal: Doctors probe why it's hard for many kids to get up (also Night Owls: Disorder may cause teens to sleep less):
Technology keeps teens awake, study shows:
Only 40 winks stinks Sleep deprivation hampers youths' growth, learning:
The science of lost sleep in teens:
New survey sounds the alarm: Nation's kids need more sleep:
Survey finds most teens are sleep-deprived:
The science of lost sleep in teens:
Just too wired: Why teens don't get enough sleep:
Studies by Brown, Lifespan scientists are at cutting edge of sleep research:
(Also, see my take on this isssue)
"The parents get stigmatized as not having control over their kids when they can't get them to school in time," says James Wyatt, co-director of the sleep-disorders center at Rush University Medical Center in Chicago who is conducting research looking for ways to better diagnose the disorder. "Children themselves get stigmatized as avoiding school or lazy. There's a lot of baggage associated with it."
Technology keeps teens awake, study shows:
An optimal night's sleep for teens is nine hours, said Mary Carskadon, director of the E.P. Bradley Hospital Sleep center at Brown University and the other study author. But researchers found that almost half slept fewer than eight hours on school nights.
Only 40 winks stinks Sleep deprivation hampers youths' growth, learning:
As kids reach adolescence, their internal clocks tend to shift, causing them to naturally feel more alert later at night and to wake up later in the morning. This change can make it difficult for them to fall asleep before 11 p.m. In fact, more than half of high school students stay up past that hour on school nights.
Sleep experts continue to advocate for later school starting times, 9 a.m. or after, a notion that hasn't been embraced by Valley districts.
"Sending students to schools without enough sleep is like sending them to school without breakfast," said Jodi Mindell, associate director of the Sleep Disorders Center at the Children's Hospital of Philadelphia and co-chair of the sleep poll task force. "Sleep serves not only as a restorative function for the adolescents' bodies and brains, but it is also a key time when they process what they've learned during the day."
The science of lost sleep in teens:
"Our results show that the adage 'early to bed, early to rise' presents a real challenge for adolescents," says Carskadon, who directs the Bradley Hospital Sleep and Chronobiology Sleep Laboratory and is a professor of psychiatry and human behavior at Brown Medical School.
Carskadon's work has been instrumental in influencing school start times across the country. Regionally, the North Kingstown School Department in Rhode Island, North Reading Public Schools in Massachusetts, and West Hartford Public Schools in Connecticut are considering school start time changes due, in part, to research on teens and sleep.
In a study published in the November 2005 issue of the journal Sleep, Carskadon found that the "sleep pressure" rate the biological trigger that causes sleepiness slows down in adolescence and is one more explanation for why teens can't fall asleep until later at night. Carskadon's newest finding indicates that, in addition to the changes in their internal clocks, adolescents experience slower sleep pressure, which may contribute to an overall shift in teen sleep cycles to later hours.
New survey sounds the alarm: Nation's kids need more sleep:
At least one of the reasons adolescents don't get enough sleep is not their fault, said Mindell. Hormone changes cause a two-hour shift in circadian rhythm (the body's internal clock), Mindell said. So they are naturally more alert later in the day.
Survey finds most teens are sleep-deprived:
Nature provides at least a partial excuse. When children reach adolescence, their circadian rhythms, or internal clocks, shift, making them more awake at night. Sixth-graders got 8.4 hours on school nights, the survey found. By contrast, seniors in high school slept an average of 6.9 hours on school nights.
The science of lost sleep in teens:
The report indicated that adolescents aged 13 to 22 need nine to 10 hours of sleep each night. It also discussed the hormonal changes that conspire against them. When puberty hits, the body's production of sleep-inducing melatonin is delayed, making an early bedtime biologically impossible for most teens. At the same time, the report notes, external forces such as after-school sports and jobs and early school start times put the squeeze on a full night's sleep.Teens rack up sleep debt:
The result: A "profound negative effect" on mood, school performance and cognitive function. Studies also show that young people between 16 and 29 years of age were the most likely to be involved in crashes caused by the driver falling asleep.
Most teens miss sleep because of the normal changes of adolescence, compounded by distraction. Sleep specialists speak of circadian rhythms, or the body's internal clock. As children reach their teens, that clock shifts: They are wider awake later at night, and prone to sleep later in the morning. This "phase delay" makes it difficult to fall asleep before 11 p.m.Sleep-Deprived Teens Paying Price:
School schedules, on the other hand, are built around busing needs and plans for daylight sports events, among other things. Most teens must get up around 6:30 or 7 a.m. to make it to school on time.
This leads to epic early-morning battles.
"In the competition between the natural tendency to stay up late and early school start times, a teen's sleep is what loses out," said Jodi A. Mindell of St. Joseph's University in Philadelphia.America's Sleep-Deprived Teens Nodding Off at School, Behind the Wheel, New National Sleep Foundation Poll Finds:
------------------------
Some school districts, most notably the Minneapolis public school district, have been trying later start times for high schools, Assuras reports. Researchers have seen some benefits, but in many school districts, conflicts with bus schedules and after-school activities make such changes extremely difficult.
Assuras says, short of getting school start times changed, teens might want to take some advice from experts, who recommend saying goodbye to the entertainment zone in the bedroom, cutting out caffeine after lunchtime, setting up a daily sleep and wake-time routine, and being aware that trying to catch up by sleeping in on weekends throws off your natural body rhythm.
As children reach adolescence, their circadian rhythms -– or internal clocks -– tend to shift, causing teens to naturally feel more alert later at night and wake up later in the morning. A trick of nature, this "phase delay" can make it difficult for them to fall asleep before 11 p.m.; more than one-half (54 percent) of high school seniors go to bed at 11 p.m. or later on school nights. However, the survey finds that on a typical school day, adolescents wake up around 6:30 a.m. in order to go to school, leaving many without the sleep they need.
"In the competition between the natural tendency to stay up late and early school start times, a teen's sleep is what loses out," notes Jodi A. Mindell, PhD, co-chair of the poll task force and an NSF vice chair. "Sending students to school without enough sleep is like sending them to school without breakfast. Sleep serves not only a restorative function for adolescents' bodies and brains, but it is also a key time when they process what they've learned during the day." Dr. Mindell is the director of the Graduate Program in Psychology at Saint Joseph's University and associate director of the Sleep Center at The Children's Hospital of Philadelphia.
Just too wired: Why teens don't get enough sleep:
The researchers said that as children reach puberty, their body clocks tended to readjust to start two hours later, so they are more alert at night and sleepier in the early morning. Mindell and Carskadon said schools should adjust their schedules accordingly, and start later. A few have done so, they said, and found that instead of staying up later, students sleep longer.
Studies by Brown, Lifespan scientists are at cutting edge of sleep research:
"Some of our kids are literally sleep-walking through life, with some potentially serious consequences," Millman said. "As clinicians and researchers, we know more now than ever about the biological and behavioral issues that prevent kids from getting enough sleep.
(Also, see my take on this isssue)
Monday, March 27, 2006
Does circadian clock regulate clutch-size in birds? A question of appropriatness of the model animal.
The questions of animal models
There are some very good reasons why much of biology is performed in just a handful of model organisms. Techniques get refined and the knowledge can grow incrementally until we can know quite a lot of nitty-gritty details about a lot of bioloigcal processes. One need not start from Square One with every new experiment with every new species. One should, of course, occasionally test how generalizable such findings are to other organisms, but the value of models is hard to dispute.
Which organisms have been chosen as laboratory models often depends on contingencies of history. Somebody at some point in time had a large supply of a particular species, did some good research on it and everything took off from there. Some species proved untractable and were discarded. Others flourished and were adopted by more and more researchers over time. Some models are really good for studying particular areas of biology. The fruitfly is unparalleled as the organism for genetic studies. However, its development is quite unusual so the findings are difficult to generalize even to other Dipterans, even less to other insects, arthropods or other animals.
What makes a good laboratory animal model?
A good animal model should be one about which there is quite a lot of background information. It should be available in large numbers and it should be rather small in size so a lab can keep large numbers of individuals in a relatively small space. Being social also makes housing easier. A good model animal is also easy to keep in captivity, it is easy to feed and easy to handle without too much danger to the technicians. Ideally, it easily breeds in captivity, has a short generation time and large numbers of progeny.
A brief aside about mammalian models
It is not surprising that early studies in anatomy and physiology utilized domesticated animals. Much of 18th and 19th century research was done in dogs, cats, rabbits, sheep, goats, pigs and even horses. Such animals were easily available, there was a lot of existing knowledge about them, and they were easy to house, feed and handle. Furthermore, one could always "sell" such research as useful for advances in agriculture. However, there are definite drawbacks in using these species as well. They tend to be large, with long generation times and with few progeny. Also, being human companions for thousands of years, people get easily attached to them and the anti-vivisectionist movement was quite strong in the 19th century, especially in England.
Thus, after several decades of effort, several new species were domesticated in order to provide the researchers with more tractable and less controversial animals to work with. These include rats, mice, hamsters, guinea pigs, ground squirrels and ferrets. Much of the research in the 20th century was performed on these species. In the last couple of decades, one of those species gained prominence - the mouse - due to its tractability to genetic techniques. Much of the findings from rodent (including mouse) studies have been found to be generalizable to other rodents, to other mammals (including humans) and to other vertebrates. Much has been learned that proved applicable to endangered species and their reproduction in captivity. Rarely is any work done on rodents these days that as its main aim has the better understanding of the rodents. Mainly, the mouse and the rat are the stand-ins for humans and the research is thought of as biomedical research.
Avian models
The bird models have quite a different history. The readily available species are the domesticated birds, better known as poultry - chicken, quail, duck, turkey, goose, pigeon and the guinea hen. There was never a need to move away from these species, as they are small, social, breed fast and a lot, and are viewed as food (and not as "cute pets") thus they were a less likely target of anti-vivisectionists.
Sure, many biologists studied wild species in the field and in the lab. Even today, much work is done in parrots, European starlings, house sparrows, house finches, crows, etc. But wild species are tough to work with. One has to go out and catch a new batch for each new experiment. Poultry is so much easier to handle and breed in the lab.
The lack of a historical "switch" from one group of species to another had one unfortunate consequence. Research on domesticated species of birds is too easily dismissed as Poultry Science, geared towards increased meat and egg production at farms (what's wrong with that? The findings from such research usually make the life for farm birds better, e.g., less stressful). It is sometimes forgotten that many scientists use poultry species with a different motivation altogether - as a model for wild birds, hopefully generalizable even broader to other vertebrates or even all animals. Many findings from poultry research are now being used to help endangered bird species survive and breed in the wild and in captivity.
Why such a long intro?
Because my lab animal is a domesticated bird, poultry if you wish - the Japanese quail. I will not be unhappy if my research gets picked up by poultry scientists and applied to make the life of farm birds easier, but that is not my main motivation. I am interested in particular aspects of basic science of chronobiology and I found quail to be an excellent model for questions that are difficult or even impossible to do in mammals. I hope that my work is generalizable to wild birds and will help in saving endangered species from extinction. I hope that at least some of my work will be even more broadly generalizable, perhaps to all vertebrates, or even to all of life.
Japanese quail (Coturnix japonica) has been domesticated about 500 years ago in Japan. The name 'Japanese quail' is used to denote the domesticated birds in laboratories and on farms. Their counterpart in the wild, itself not an endangered species, is usually called Asian Migratory Quail, and it appears that there is not that much difference between wild and domesticated populations in many aspects of their biology. With incubation time of 17 days, maturation time of six weeks, and ability to lay almost an egg a day throughout the year, quail is the mouse of the avian biology lab. The chicken genome has been sequenced. Every gene that has beem looked at is between 95 and 100% identical between the chicken and the quail. It appears that the difference between the two species is mainly due to regulatory regions of the genes driving somewhat different trajectoris of embryonic developments, similarly to the difference between humans and chimps.
An important part of my Masters degree research (but not my PhD) was on the mechanisms by which the circadian system controls reproduction - both seasonally (photoperiodism) and daily (the timing of egg-laying). My MS thesis has been published in these two papers [Note: the first one appears not to be available in its entirety online - you can only see the abstract. I have notified the Journal and once this is fixed I will make the appropriate link to the PDF of the whole paper. Update: The paper is now online and can be accessed here].
Circadian control of egg-laying
Circadian system in Japanese quail, and presumably in many other (perhaps all?) species of birds, is a two-oscillator system. It is as if the brain contains two separate circadian clocks, each with its own properties. These two clocks also interact with each other. One of the clocks is easily and directly entrained by the environmental light-dark cycles. It governs the overt daily rhythms of such things as body temperature, feeding activity, heart rate, blood pressure and release of hormones such as melatonin. The other clock appears not to be directly sensitive to light. It seems that it gets its information about the environmental lighting conditions from its interaction with the first clock. This second clock (or second component of the circadian system) also drives rhythms such as activity and body temperature, but more importantly, it drives the circadian rhythms of ovulation and oviposition. In this species, once an egg is laid, the next follicle ovulates about 45 minutes later. It takes anywhere between 24 and 30 hours for an ovulated egg to be laid. Thus, one egg is laid each day.
The two circadian clocks interact in ways that are possible to describe using models borrowed from the physics of oscillations (remember the pendulum from high school?). One of the predictions from the model is that some biological events can happen only when the two oscillators are in a particular phase-relationship with each other. This phase, in regards to ovulation in birds, is sometimes called "the permissive zone". Only if the ovary is ready to ovulate during this zone, ovulation will occur. Otherwise, it will wait until the next day.
In short daylengths, e.g., during the winter, the permissive zone is so narrow (or even non-existent), so that no ovulation happens at all. The bar on top of the graph indicates the duration of the day - white and night - black. The first line is first day, the line below it is the second day, etc. X-axis is 24 hours long. Bluish area shows the times when the first clock drives body temperature to be above the daily mean and drives the animal to be behaviorally active; the white area shows the times when the first clock determines when the quail is asleep and the melatonin levels are high.
As the photoperiod increases in spring, the permissive zone appears (between two dotted lines) and is broad enough that ovulation can happen. In relatively short photoperiods (but above the critical photoperiod for the onset of ovulation), the two clocks are quite tightly coupled. They are almost entrained to each other - they exhibit what is called relative coordination, which means that the two clocks can entrain ("lock") to each other for a few cycles but cannot remain phase-locked indefinitely:
The first egg gets laid at the beginning of the permissive phase. Each day, the second clock tries to escape from the pull of the first clock and to time the egg-laying a little bit later. Finally, the ovulation reaches the end of the permissive phase and the clutch stops. If everything is OK, prolactin will kick in to prevent the hen from laying any more eggs and she can sit down on her eggs and incubate them. If a predator, like a snake, eats the eggs (or a researcher removes the eggs from the cage) there is no rise in prolactin and the quail starts a new clutch all over again, following the same pattern, after one or two days of pause.
A couple of months later, after the bird has succesfully raised a brood of hatchlings, the quail may decide to renest. This is now early summer and the daylength is much longer. The permissive phase is much broader and intrudes even into the night. On the other hand, the coupling between the two clocks is now very weak and the second clock freeruns with its own inherent period which can be anywhere between 26 and 30 hours. The pattern of egg-laying now looks something like this:
Now compare the last two figures. One thing that you will notice is that the clutch consisted of more eggs in shorter photoperiod (spring), than in the long photoperiod (summer). This is something that has been observed in a number of species of birds in the wild. The above figures are schematics. Here are some real data. Every 10-minute period that is black denotes the time when body temperature is above the daily mean. The light bars on top show when the lighs were on (white) and off (black). Circles show times when eggs were laid. On the top is a quail in LD 14:10. In the middle is a quail in LD 18:6. On the bottom are two examples of oviposition patterns during a transition from LD 14:10 to LD 18:6 (all from [1]):
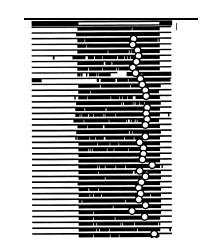
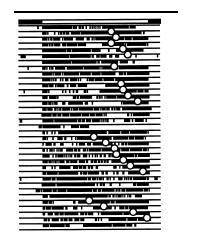
Not so obvious, but the time-difference between two successive eggs in spring is just a little bit longer than 24 hours. In summer, this difference is 26-30 hours. This means that in summer, each follicle has more time to get filled by filtering of blood and each egg has more time to spend in the oviduct and the shell-gland. One should expect that summer eggs will be, on average, larger, heavier and with thicker shell than eggs laid in early spring. Evolutionary theory predicts the existence of a trade-off between egg-number (i.e., clutch-size) and egg-size. It is almost like a switch between r-strategy and K-strategy. In spring, premium is put on quantity and in summer on quality of progeny. The quail literature suggests that the circadian clock is the physiological mechanism underlying this switch in evolutionary strategies.
How generalizable is this finding?
Apart from Japanese quail, few other species have been studied. The sister species, European Migratory Quail (Coturnix coturnix), which is not domesticated, has virtually identical egg-laying patterns. In domestic chickens, it looks very similar, except that the first egg is laid at dawn (as opposed to noon) and the last egg is laid around noon (as opposed to evening). A couple of studies in turkeys also suggest the same mechanism.
Can we generalize this finding to all Gallinaceous birds at least? Sounds reasonable, but I do not know. Is the same mechanism operating in birds from other Orders? Hummingbirds? Owls? Ostriches?
There is an interaction between estrous cycle and circadian cycle in rodents. Sex steroid hormones that are released during ovulation have effects on circadian clocks in several species of birds and mammals (presumably also humans). Does this information make it seem more likely that the quail data are generalizable to all birds?
Every time I ask a friend who studies wild birds at what times the eggs are laid, the answer is "I don't know - we usually find the eggs in the morning". This means that the egg could have been laid at any time between 5pm and 9am - a very broad region. No temporal patterns appear to be known in wild birds. So, if you study wild birds, please let me know at what time of day the eggs are laid. Let's see if the data gathered from poultry are useful for the study of wild species as well.
[1] Bora D. Zivkovic, Herbert Underwood, and Thomas Siopes, Circadian Ovulatory Rhythms in Japanese Quail: Role of Ocular and Extraocular Pacemakers, JOURNAL OF BIOLOGICAL RHYTHMS, Vol. 15 No. 2, April 2000 172-183
There are some very good reasons why much of biology is performed in just a handful of model organisms. Techniques get refined and the knowledge can grow incrementally until we can know quite a lot of nitty-gritty details about a lot of bioloigcal processes. One need not start from Square One with every new experiment with every new species. One should, of course, occasionally test how generalizable such findings are to other organisms, but the value of models is hard to dispute.
Which organisms have been chosen as laboratory models often depends on contingencies of history. Somebody at some point in time had a large supply of a particular species, did some good research on it and everything took off from there. Some species proved untractable and were discarded. Others flourished and were adopted by more and more researchers over time. Some models are really good for studying particular areas of biology. The fruitfly is unparalleled as the organism for genetic studies. However, its development is quite unusual so the findings are difficult to generalize even to other Dipterans, even less to other insects, arthropods or other animals.
What makes a good laboratory animal model?
A good animal model should be one about which there is quite a lot of background information. It should be available in large numbers and it should be rather small in size so a lab can keep large numbers of individuals in a relatively small space. Being social also makes housing easier. A good model animal is also easy to keep in captivity, it is easy to feed and easy to handle without too much danger to the technicians. Ideally, it easily breeds in captivity, has a short generation time and large numbers of progeny.
A brief aside about mammalian models
It is not surprising that early studies in anatomy and physiology utilized domesticated animals. Much of 18th and 19th century research was done in dogs, cats, rabbits, sheep, goats, pigs and even horses. Such animals were easily available, there was a lot of existing knowledge about them, and they were easy to house, feed and handle. Furthermore, one could always "sell" such research as useful for advances in agriculture. However, there are definite drawbacks in using these species as well. They tend to be large, with long generation times and with few progeny. Also, being human companions for thousands of years, people get easily attached to them and the anti-vivisectionist movement was quite strong in the 19th century, especially in England.
Thus, after several decades of effort, several new species were domesticated in order to provide the researchers with more tractable and less controversial animals to work with. These include rats, mice, hamsters, guinea pigs, ground squirrels and ferrets. Much of the research in the 20th century was performed on these species. In the last couple of decades, one of those species gained prominence - the mouse - due to its tractability to genetic techniques. Much of the findings from rodent (including mouse) studies have been found to be generalizable to other rodents, to other mammals (including humans) and to other vertebrates. Much has been learned that proved applicable to endangered species and their reproduction in captivity. Rarely is any work done on rodents these days that as its main aim has the better understanding of the rodents. Mainly, the mouse and the rat are the stand-ins for humans and the research is thought of as biomedical research.
Avian models
The bird models have quite a different history. The readily available species are the domesticated birds, better known as poultry - chicken, quail, duck, turkey, goose, pigeon and the guinea hen. There was never a need to move away from these species, as they are small, social, breed fast and a lot, and are viewed as food (and not as "cute pets") thus they were a less likely target of anti-vivisectionists.
Sure, many biologists studied wild species in the field and in the lab. Even today, much work is done in parrots, European starlings, house sparrows, house finches, crows, etc. But wild species are tough to work with. One has to go out and catch a new batch for each new experiment. Poultry is so much easier to handle and breed in the lab.
The lack of a historical "switch" from one group of species to another had one unfortunate consequence. Research on domesticated species of birds is too easily dismissed as Poultry Science, geared towards increased meat and egg production at farms (what's wrong with that? The findings from such research usually make the life for farm birds better, e.g., less stressful). It is sometimes forgotten that many scientists use poultry species with a different motivation altogether - as a model for wild birds, hopefully generalizable even broader to other vertebrates or even all animals. Many findings from poultry research are now being used to help endangered bird species survive and breed in the wild and in captivity.
Why such a long intro?
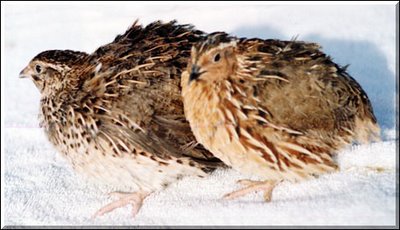
Japanese quail (Coturnix japonica) has been domesticated about 500 years ago in Japan. The name 'Japanese quail' is used to denote the domesticated birds in laboratories and on farms. Their counterpart in the wild, itself not an endangered species, is usually called Asian Migratory Quail, and it appears that there is not that much difference between wild and domesticated populations in many aspects of their biology. With incubation time of 17 days, maturation time of six weeks, and ability to lay almost an egg a day throughout the year, quail is the mouse of the avian biology lab. The chicken genome has been sequenced. Every gene that has beem looked at is between 95 and 100% identical between the chicken and the quail. It appears that the difference between the two species is mainly due to regulatory regions of the genes driving somewhat different trajectoris of embryonic developments, similarly to the difference between humans and chimps.
An important part of my Masters degree research (but not my PhD) was on the mechanisms by which the circadian system controls reproduction - both seasonally (photoperiodism) and daily (the timing of egg-laying). My MS thesis has been published in these two papers [Note: the first one appears not to be available in its entirety online - you can only see the abstract. I have notified the Journal and once this is fixed I will make the appropriate link to the PDF of the whole paper. Update: The paper is now online and can be accessed here].
Circadian control of egg-laying
Circadian system in Japanese quail, and presumably in many other (perhaps all?) species of birds, is a two-oscillator system. It is as if the brain contains two separate circadian clocks, each with its own properties. These two clocks also interact with each other. One of the clocks is easily and directly entrained by the environmental light-dark cycles. It governs the overt daily rhythms of such things as body temperature, feeding activity, heart rate, blood pressure and release of hormones such as melatonin. The other clock appears not to be directly sensitive to light. It seems that it gets its information about the environmental lighting conditions from its interaction with the first clock. This second clock (or second component of the circadian system) also drives rhythms such as activity and body temperature, but more importantly, it drives the circadian rhythms of ovulation and oviposition. In this species, once an egg is laid, the next follicle ovulates about 45 minutes later. It takes anywhere between 24 and 30 hours for an ovulated egg to be laid. Thus, one egg is laid each day.
The two circadian clocks interact in ways that are possible to describe using models borrowed from the physics of oscillations (remember the pendulum from high school?). One of the predictions from the model is that some biological events can happen only when the two oscillators are in a particular phase-relationship with each other. This phase, in regards to ovulation in birds, is sometimes called "the permissive zone". Only if the ovary is ready to ovulate during this zone, ovulation will occur. Otherwise, it will wait until the next day.
In short daylengths, e.g., during the winter, the permissive zone is so narrow (or even non-existent), so that no ovulation happens at all. The bar on top of the graph indicates the duration of the day - white and night - black. The first line is first day, the line below it is the second day, etc. X-axis is 24 hours long. Bluish area shows the times when the first clock drives body temperature to be above the daily mean and drives the animal to be behaviorally active; the white area shows the times when the first clock determines when the quail is asleep and the melatonin levels are high.
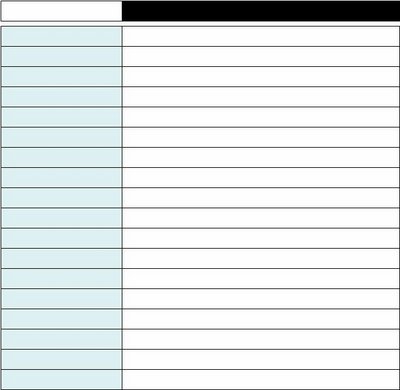
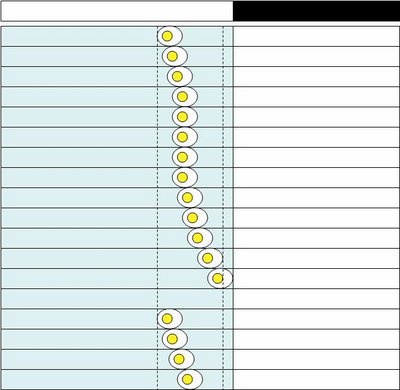
A couple of months later, after the bird has succesfully raised a brood of hatchlings, the quail may decide to renest. This is now early summer and the daylength is much longer. The permissive phase is much broader and intrudes even into the night. On the other hand, the coupling between the two clocks is now very weak and the second clock freeruns with its own inherent period which can be anywhere between 26 and 30 hours. The pattern of egg-laying now looks something like this:

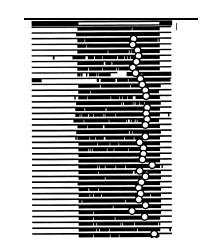
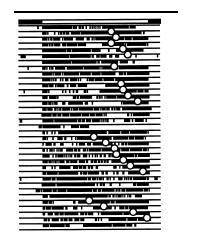
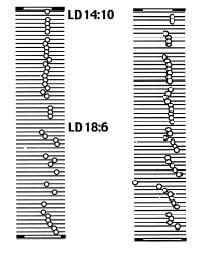
How generalizable is this finding?
Apart from Japanese quail, few other species have been studied. The sister species, European Migratory Quail (Coturnix coturnix), which is not domesticated, has virtually identical egg-laying patterns. In domestic chickens, it looks very similar, except that the first egg is laid at dawn (as opposed to noon) and the last egg is laid around noon (as opposed to evening). A couple of studies in turkeys also suggest the same mechanism.
Can we generalize this finding to all Gallinaceous birds at least? Sounds reasonable, but I do not know. Is the same mechanism operating in birds from other Orders? Hummingbirds? Owls? Ostriches?
There is an interaction between estrous cycle and circadian cycle in rodents. Sex steroid hormones that are released during ovulation have effects on circadian clocks in several species of birds and mammals (presumably also humans). Does this information make it seem more likely that the quail data are generalizable to all birds?
Every time I ask a friend who studies wild birds at what times the eggs are laid, the answer is "I don't know - we usually find the eggs in the morning". This means that the egg could have been laid at any time between 5pm and 9am - a very broad region. No temporal patterns appear to be known in wild birds. So, if you study wild birds, please let me know at what time of day the eggs are laid. Let's see if the data gathered from poultry are useful for the study of wild species as well.
[1] Bora D. Zivkovic, Herbert Underwood, and Thomas Siopes, Circadian Ovulatory Rhythms in Japanese Quail: Role of Ocular and Extraocular Pacemakers, JOURNAL OF BIOLOGICAL RHYTHMS, Vol. 15 No. 2, April 2000 172-183
Sunday, March 26, 2006
Sleep Schedules in Adolescents
I am glad to see that there is more and more interest in and awareness of sleep research. Just watch Sanjay Gupta on CNN or listen to the recent segment on Weekend America on NPR.
At the same time, I am often alarmed at the levels of ignorance still rampant in the general population, and even more the negative social connotations of sleep as an indicator of laziness.
Nothing pains me more than when I see educators (in comments) revealing such biases in regards to their student in the adolescent years. Why do teachers think that their charges are lazy, irresponsible bums, and persist in such belief even when confronted with clear scientific data demonstrating that sleep phase in adolescents is markedly delayed in comparison to younger and older people?
The shift in sleep-phase of adolescents is one of the best documented and most studied phenomena in human chronobiology. If you dig through my ClockNews category, you'll see that almost every issue has something about adolescent sleep patterns. My first and still most popular post here addresses this phenomenon in some detail (as well as some advice), especially this sweet paper that came out a couple of years ago (follow the references within or search MedLine, Web of Science or Google Scholar for more information).
In short, presumably under the influence of the sudden surge of sex steroid hormones (and my own research gently touches on this), the circadian clock phase-advances in teen years. It persists in this state until one is almost 30 years old. After that, it settles into its adult pattern. Of course, we are talking about human populations - you can surely give me an anecdote about someone who does not follow this pattern. That's fine. Of course there are exceptions, as there is vast genetic (and thus phenotypic) variation in human populations. This does not in any way diminish the findings of population studies.
Everyone, from little children, through teens and young adults to elderly, belongs to one of the 'chronotypes'. You can be a more or less extreme lark (phase-advanced, tend to wake up and fall asleep early), a more or less extreme owl (phase-delayed, tend to wake up and fall asleep late). You can be something in between - some kind of "median" (I don't want to call this normal, because the whole spectrum is normal) chronotype.
Along a different continuum, one can be very rigid (usually the extreme larks find it really difficult to adjust to work schedules that do not fit their clocks), or quite flexible (people who find it easy to work night-shifts or rotating shifts and tend to remain in such jobs long after their colleagues with less flexible clocks have quit).
No matter where you are on these continua, once you hit puberty your clock will phase-delay. If you were an owl to begin with, you will become a more extreme owl for about a dozen years. If you are an extreme lark, you'll be a less extreme lark. In the late 20s, your clock will gradually go back to your baseline chronotype and retain it for the rest of your life.
The important thing to remember is that chronotypes are not social constructs (although work-hours and school-hours are). No amount of bribing or threatening can make an adolescent fall asleep early. Don't blame video games or TV. Even if you take all of these away (and you should that late at night, and replace them with books) and switch off the lights, the poor teen will toss and turn and not fall asleep until midnight or later, thus getting only about 4-6 hours of sleep until it is time to get up and go to school again.
More and more school districts around the country, especially in more enlightened and progressive areas are heeding the science and making a rational decision to follow the science and adjust the school-start times accordingly. Instead of forcing teenagers to wake up at their biological midnight (circa 6am) to go to school, where invariably they sleep through the first two morning classes, more and more schools are adopting the reverse busing schedule: elementary schools first (around 7:50am), middle schools next (around 8:20am) and high schools last (around 8:50am). I hope all schools around the country eventually adopt this schedule and quit torturing the teens and then blaming the teens for sleeping in class and making bad grades.
No matter how much you may wish to think that everything in human behavior originates in culture, biology will trump you every now and then, and then you should better pay attention, especially if the life, health, happiness and educational quality of other people depends on your decisions.
(See more here)
At the same time, I am often alarmed at the levels of ignorance still rampant in the general population, and even more the negative social connotations of sleep as an indicator of laziness.
Nothing pains me more than when I see educators (in comments) revealing such biases in regards to their student in the adolescent years. Why do teachers think that their charges are lazy, irresponsible bums, and persist in such belief even when confronted with clear scientific data demonstrating that sleep phase in adolescents is markedly delayed in comparison to younger and older people?
The shift in sleep-phase of adolescents is one of the best documented and most studied phenomena in human chronobiology. If you dig through my ClockNews category, you'll see that almost every issue has something about adolescent sleep patterns. My first and still most popular post here addresses this phenomenon in some detail (as well as some advice), especially this sweet paper that came out a couple of years ago (follow the references within or search MedLine, Web of Science or Google Scholar for more information).

Everyone, from little children, through teens and young adults to elderly, belongs to one of the 'chronotypes'. You can be a more or less extreme lark (phase-advanced, tend to wake up and fall asleep early), a more or less extreme owl (phase-delayed, tend to wake up and fall asleep late). You can be something in between - some kind of "median" (I don't want to call this normal, because the whole spectrum is normal) chronotype.
Along a different continuum, one can be very rigid (usually the extreme larks find it really difficult to adjust to work schedules that do not fit their clocks), or quite flexible (people who find it easy to work night-shifts or rotating shifts and tend to remain in such jobs long after their colleagues with less flexible clocks have quit).
No matter where you are on these continua, once you hit puberty your clock will phase-delay. If you were an owl to begin with, you will become a more extreme owl for about a dozen years. If you are an extreme lark, you'll be a less extreme lark. In the late 20s, your clock will gradually go back to your baseline chronotype and retain it for the rest of your life.
The important thing to remember is that chronotypes are not social constructs (although work-hours and school-hours are). No amount of bribing or threatening can make an adolescent fall asleep early. Don't blame video games or TV. Even if you take all of these away (and you should that late at night, and replace them with books) and switch off the lights, the poor teen will toss and turn and not fall asleep until midnight or later, thus getting only about 4-6 hours of sleep until it is time to get up and go to school again.
More and more school districts around the country, especially in more enlightened and progressive areas are heeding the science and making a rational decision to follow the science and adjust the school-start times accordingly. Instead of forcing teenagers to wake up at their biological midnight (circa 6am) to go to school, where invariably they sleep through the first two morning classes, more and more schools are adopting the reverse busing schedule: elementary schools first (around 7:50am), middle schools next (around 8:20am) and high schools last (around 8:50am). I hope all schools around the country eventually adopt this schedule and quit torturing the teens and then blaming the teens for sleeping in class and making bad grades.
No matter how much you may wish to think that everything in human behavior originates in culture, biology will trump you every now and then, and then you should better pay attention, especially if the life, health, happiness and educational quality of other people depends on your decisions.
(See more here)
Jet-lag is bad for you long-term, too
Chronic 'jet lag' produces temporal lobe atrophy and spatial cognitive deficits:
Hat-tip: Eide Neurolearning Blog
Time-zone travelers encounter a pattern of light and darkness, and their endogenous circadian rhythms adapt to the new external time cue until both timing systems synchronize, but the long-term repeated disturbance of synchronization between the two timing systems impairs physiological and psychological health and induces stress. Salivary cortisol levels in cabin crew after repeated exposure to jet lag were significantly higher than after short distance flights, and the higher cortisol levels were associated with cognitive deficits that were dependent on non-semantic stimuli. The present study demonstrates that significant prolonged cortisol elevations produce reduced temporal lobe volume and deficits in spatial learning and memory; these cognitive deficits became apparent after five years of exposure to high cortisol levels.
Hat-tip: Eide Neurolearning Blog
Sunday, March 19, 2006
Some hypotheses about a possible connection between malaria and jet-lag
Malaria Action Day is today, March 19th. Read Tara, Tim and me for more information. You should play basketball today, as part of the Dunk Malaria initiative. You should donate to The Global Fund for the fight against malaria (and send Tim the confirmation e-mail you get, so he can match it up to $300). Post this information on your blog (or e-mail to friends) today. Write a blog-post or journal entry about malaria or something related and I will put together a linkfest on Sunday (on Science And Politics), linking to all bloggers who send me permalinks of their posts on the topic. If you have not written anything recently, but have a good post from the past, send it anyway. The posts need not focus on biology or medicine of malaria - writings on history, geography, economics and politics of this disease are equally welcome.
I have written a little bit about malaria before, e.g, here and here, but this is my special Malaria Action Day post, inspired by a paper [1] that Tara sent me some weeks ago and I never got to write about it till now.
-------------------------------------------------------------------------------
In a journal called "Medical Hypotheses" Kumar and Sharma [1] propose that jet-lagged travellers may be more susceptible to getting infected with malaria. They write:
1) affect the rate/ease of infection with malaria,
2) affect the symptoms of malaria in an infected individual,
3) affect the ability of the body to fight off the infection,
4) affect the effectiveness of treatments, and
5) affect the likelihood that the infected individual will spread the disease to others.
The Kumar/Sharma hypothesis is clearly of the #1 type. I will look more at other types of hypotheses - those that apply to already infected individuals. For that, let's first go quickly through the basic biology of malaria.
Malaria is caused by a protist in the genus Plasmodium. While Plasmodium falciparum is the most common species, three or four other species are also causes of malaria in humans, and dozens of other species cause malaria or malaria-like diseases in other animals, including mammals, birds and reptiles.
Plasmodium is transmitted through bites of several species of mosquito from the genus Anopheles. Once injected into the final host (e.g., human), the plasmodia remain in the skin for several hours, then migrate to lymph nodes, spleen and liver where they undergo several transformations. The final stage - the gametocyte - migrates into the red blood cells. Inside each red blood cell one can find a large number of plasmodia, hiding there from the immune system of the host. The whole life-cycle lasts several days, even weeks to complete.
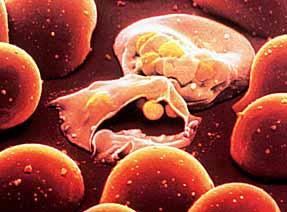
All the plasmodia burst out of red blood cells simultaneously. Enormous number of plasmodia suddenly released into the blood overwhelms the immune system of the host, allowing the plasmodia to survive unscathed for quite a long time. This time is sufficient for them to invade blood vessels in the skin where, if they are lucky, a mosquito will bite and the plasmodia can invade the mosquito again and search for the next host.
The bursting of red blood cells triggers high fever and sweating. High temperature, high carbon-dioxide, as well as some odors [2] present in the sweat are all highly attractive to mosquitoes, rasing the probability that the host will get bitten. In some species of Plasmodium (like P.falciparum), the bursting of red blood cells occurs every night. In some species of Plasmodium, the resulting fever occurs every two nights and in some every four nights (rarely three), causing, respectively, tertian and quartan fevers. Tertian and quartan malaria are treated by chloroquine, while falciparum malaria is treated by quinine, mefloquine or halofantrine.
Obviously, from the perspective of a Plasmodium, timing is crucial. First, it is important to errupt in synchrony. Yet, hidden inside red blood cells, plasmodia cannot communicate with each other. Second, it is important to time the eruption in such a way as to maximize the probabilty that some of the gametocytes will be picked up by mosquitoes. Thus, it is important for the eruption to occur at the time of day when mosquitoes are most actively foraging for blood.
How do the Plasmodia solve the problem of timing? This is where circadian biology comes in [3,4,5]. Plasmodia residing inside red blood cells use the time-clues generated by the host. More specifically, they key onto the nightly release of melatonin by the pineal gland. Melatonin is practically undetectable in the blood during the day and the concentrations rise steeply in the evening remaining high for the duration of the night (exact patterns differ between vertebrate species), then dropping again at dawn.
Plasmodia have melatonin receptors [3]. Interestingly, unlike melatonin receptors in vertebrates which are nuclear receptors, the receptors in Plasmodia are membrane receptors. Membrane receptors are much faster than nuclear receptors which is important when a biological event has to be timed with precision. However, the plasmodia do not destroy the red blood cell immediately after receiving the melatonin signal - that would be too early in the evening for the timing to be adaptive, as the mosquitoes are still too busy looking for mates and mating at that time. Instead, the plasmodia use their own circadian clocks to measure the exact timing of eruption. In a way, it appears that the host melatonin signal entrains (synchronizes) the clocks in plasmodia, and then the Plasmodium clock determines the phase (exact timing) for the eruption out of red blood cells.
Different species of Anopheles and even geographically distinct populations of the same species have different times of peak foraging (biting) activity. In each geographical region, the local population (or species) of Plasmodium evolved the timing of eruption to match that of the local mosquitoes.
Let's now introduce another player. Apart from the parasite (Plasmodium), the host (a vertebrate, e.g., a human), and the vector (mosquito), one should also consider the predator - insectivorous bats that hunt for mosquitoes. The way that the malaria literature tends to think about timing can schematically be presented like this:
There is an assumption that plasmodium eruption, human fever, mosquito foraging and bat hunting are all synchronous. We have already looked at this from the perspective of the Plasmodium - it is adaptive for the Plasmodium for the three bottom lines to be accurate, i.e, that the parasite, the host and the vector are in synchrony. This also means that this is maladaptive to humans. It is also maladaptive to mosquitoes whose fitness does suffer somewhat if they are loaded with parasites.
On the other hand, it is maladaptive for mosquitoes and plasmodia, and adaptive for humans and bats, if the peak hunting time for bats coincides with the peak foraging time of mosquitoes. More these two events are in sync, more mosquitoes will get eaten, thus less plasmodia will get into a new host and less humans will get infected.
The dynamics of the timing relationship between the four species can be described as an Evolutionary Arms-Race Around The Circadian Clock. While some of the players will try to maximize their fitness by achieving synchrony, the other players maximize their fitness by avoiding synchrony with each other. This can be depicted, for bats and mosquitoes, like this:
In this case, mosquitoes evolve to forage at later times of night, and bats evolve to track the mosquitoes by hunting later at night. This can go on back and forth endlessly. But, and here is a big "but". This model is quite oversimplified as it posits only four players and for each player an absolute loyalty to the other three. But is the real world that simple?
Plasmodium species are pretty host-specific. Species that thrive inside humans, may not thrive or even survive inside the bodies of other animals and vice versa. So, the parasite is pretty loyal to its host. It is also completely dependent on Anopheles - it will most likely not survive inside a different kind of mosquito.
The same mosquito that usually bites a human will happily take a blood meal from another animal. This is actually used as one of the prevention techniques: a village is surrounded by fields full of cattle, sheep, goats, horses, donkeys or camels. The mosquitoes coming out of the woods at night encounter these animals first and get satiated with blood before they ever encounter humans. The animals themselves do not get sick.
Bats are unlikely, in my opinion, to be specialized on Anopheles as their only prey. If there are no mosquitoes around, they will happily hunt other insects (and the tropical regions where malaria is common are swarming with many species of insects!). I think that involvement of bats in the arms-race is the weakest aspect of the hypothesis. Here are four basic types of bat hunting activity that are theoretically possible:
The hypothesis suggests that bats mostly fly around midnight when the mosquitoes are most active, i.e., the bats are winners and mosquitoes loosers in the arms-race (A) . If the peak is at some other point during the night, that would suggest that bats are involved in the arms-race but the mosquitoes are currently winning (B). This may also suggest that bats highly prefer some other type of prey. The bats may be active throughout the night (C) which seems most likely. Finally, the bats may have a bimodal distribution: a lot of hunting early and late at night with a siesta right around midnight (D). This would suggest that mosquitoes have found their best temporal niche in that dangerous world, i.e, although the bats are not involved in the arms-race, the mosquitoes are and are thus winners, without making the bats "loosers" in the process.
What is the real story? I don't know. Obviously, it is possible to monitor patterns of bat activity [6,7], yet it still needs to be done in regions in which malaria is common. Some of the bats studied in the USA follow predominantly pattern C from the figure above, and it is not too far-fetched to hypothesize that all bats everywhere have similar patterns:
What are the Anopheles patterns? While they search for blood around midnight, that is not the only time they are flying around. Most of the early part of night is spent looking for mates, mating and laying eggs [8]. Thus, they are easy pickings for bats at times when they are not actively seeking humans. It appears that becoming diurnal is not a good option for Anopheles in the tropics - perhaps there are more birds there than bats, or the birds are more dangerous? It is not impossible for a mosquito to become diurnal - the mosquito we are used to seeing around here - the Culex - is crepuscular (dawn and dusk) and the Asian tiger mosquito is fully diurnal.
How does jet-lag figure in here? Apart from the hypothesis stated by Kumar and Sharma that itch sensitivity to mosquito bites gets displaced (and what I added - that temperature rhythm is also displaced), jet-lag will have other effects, too. Let's look at possible effects it may have on people who already have malaria (and you'll see why I had to use so much space describing all of the details of the arms-race above!).
Will jet-lag affect the way our body copes with the infection? In a jet-lagged human, there is no clear and sharp rhythm of melatonin release. Some amounts of melatonin are synthetized and secreted at all times of day. This means that the Plasmodium has lost its temporal anchor - there is no signal to use for determination of timing for eruption out of red blood cells. Thus, the gametocytes will errupt at random times - one cell now, another in an hour, another tomorrow. There is no safety in numbers any more - the human immune system is now perfectly capable of dealing with all the plasmodia in the circulation. Of course, the immune system itself may be somewhat compromised in a jet-lagged person.
Will jet-lag affect the way malaria presents its symptoms? The asynchronous eruption of plasmodia also means that there will be no abrupt onset of high fever at midnight. Instead, one may expect a continous low-grade fever. Nightly episodes of high fever are an important symptom of malaria. Will a physician with a patient who exhibits continuous low-grade fever ever suspect malaria? Especially a physician in a country in which there is no malaria and the patient has returned home from the tropical travels and is jet-lagged from a return trip.
Will jet-lag affect the effectiveness of drug treatments? I don't know the details of the way anti-malarial drugs work, so make sure you tell me if I get this all wrong. If the number of plasmodia in the circulation at any time is relatively small, and if the enzymatic destruction of the drug by liver is operating at a constant low rate (instead of with a circadian rhythm of its own), then being jet-lagged should enhance the effectiveness of the drugs, or even allow for the dose to be lowered.
Will jet-lag affect the ability of the patient to be a source of transmittion of the disease to others? With plasmodia erupting at all times of day and with most plasmodia being destroyed by the immune system throughout the day, it is much less likely that any will be present in the skin capillaries at just the right time - at midnight. Also, without a high fever coupled with sweating, the patient is less attractive to the mosquitoes than a malarial patient in the neighboring house who is local and not jet-lagged. Thus, the likelihood of plasmodia being picked up by mosquitoes is even smaller.
To summarize: according to the Kumar/Sharma hypothesis, being jet-lagged increases the chances for contracting malaria. On the other hand, if you already have the disease, it may be good for you to get jet-lagged! As long as you tell your physician that malaria is a serious option so the symptoms are not misinterpreted, you should be better off jet-lagged, allowing your body to fight the disease one plasmodium at a time.
Finally, as a matter of public health policy, how does one get the whole population of malarial patients in one country jet-lagged so as to reduce the transmission rates? Should hospitals induce jet-lag in malaria patients by shifting light-cycles or administering melatonin? How do the pros and cons of such treatment balance? Ah, so many hypotheses, so little data! I hope someone studies this in the future.
One last thing - notice that much of the work described above was performed by researchers outside of USA. Apart from a little bit of cellular physiology, most of the information comes from ecological field-work, and ALL of it is inspired by and informed by evolutionary theory. Not a single gel was run.
Now, I am not dissing molecular biology. Malaria is the only complex parasitic disease in which all players (plasmodium, mosquito and human) have their complete genomes sequenced, and much will be gleaned from such data in terms of designing better anti-malarial drugs, etc. But, as the above research shows, Big (molecular) Biology is not neccessary for findings that have a potential to seriously affect the infection and transmission rates of the disease.
[1] Jet lag and enhanced susceptibility to malaria, C. Jairaj Kumar and Vijay Kumar Sharma, Medical Hypotheses (2006) 66, 671–685
[2] Fooling Anopheles: Scientists Aim to Wipe Out Malaria by Outsmarting a Mosquito's Sense of Smell
[3] Calcium-dependent modulation by melatonin of the circadian rhythm in malarial parasites, Carlos T. Hotta, Marcos L. Gazarini, Flávio H. Beraldo, Fernando P. Varotti, Cristiane Lopes, Regina P. Markus, Tullio Pozzan and Célia R. S. Garcia, NATURE CELL BIOLOGY , VOL 2, JULY 2000, p.468
[4] Melatonin and N-acetyl-serotonin cross the red blood cell membrane and evoke calcium mobilization in malarial parasites, C.T. Hotta, R.P. Markus and C.R.S. Garcia, Braz J Med Biol Res 36(11) 2003
[5] Tertian and Quartan Fevers: Temporal Regulation in Malarial Infection, Célia R. S. Garcia, Regina P. Markus, and Luciana Madeira, JOURNAL OF BIOLOGICAL RHYTHMS, Vol. 16 No. 5, October 2001 436-443
[6] Sampling bats for six or twelve hours in each night? Esberard CEL, Bergallo HG, REVISTA BRASILEIRA DE ZOOLOGIA 22 (4): 1095-1098 DEC 2005
[7] Nightly, seasonal, and yearly patterns of bat activity at night roosts in the Central Appalachians, Agosta SJ, Morton D, Marsh BD, Kuhn KM, JOURNAL OF MAMMALOGY 86 (6): 1210-1219 DEC 2005
[8] Daily oviposition patterns of the African malaria mosquito Anopheles gambiae Giles (Diptera: Culicidae) on different types of aqueous substrates, Leunita A Sumba, Kenneth Okoth, Arop L Deng, John Githure, Bart GJ Knols, John C Beier and Ahmed Hassanali, Journal of Circadian Rhythms 2004, 2:6
I have written a little bit about malaria before, e.g, here and here, but this is my special Malaria Action Day post, inspired by a paper [1] that Tara sent me some weeks ago and I never got to write about it till now.
-------------------------------------------------------------------------------
In a journal called "Medical Hypotheses" Kumar and Sharma [1] propose that jet-lagged travellers may be more susceptible to getting infected with malaria. They write:
Rapid travel across several time zones leads to constellation of symptoms popularly known as "jet lag", caused primarily due to mismatch between the timing of circadian clocks of the traveller and the external periodic environment. It is often seen that the jet-lagged individuals who visit their family and friends in areas endemic to malaria have an enhanced susceptibility to malarial infection than the local residents. It would therefore be interesting to explore whether increased susceptibility to malarial infection among the visitors has anything to do with their state of jetlagged.Indeed an interesting hypothesis. Of course, the travellers may also be less resistant to malaria than the locals, or less likely to have a life-style and behavioral patterns conducive to avoiding the mosquito bites, something that may be "second nature" to the locals. They continue:
Individuals with moderate to severe skin response to mosquito bite are largely protected against mosquito borne malaria because itch alerts an individual to mosquito bite and prepares him/her to take necessary precautions to prevent mosquito bite. Itch in an individual follows a diurnal pattern, and it is about hundred folds higher during midnight than midday. A hundred fold increase in itch sensitivity is viewed as a crucial preventive measure against mosquito bites, as this coincides with the midnight flight activity peak of female Anopheles mosquitoes, when she sucks blood from the host after mating peaks in the evening to raise her progeny. Normally individuals residing in endemic areas have their daily peak of itch sensitivity overlapping with the peak – biting phase of female Anopheles mosquitoes. As a result, they are relatively well protected against malarial infection.Interesting idea: if you are sensitive to bites at the time when no mosquitoes are flying and are not sensitive to bites at the time when mosquitoes are flying, you may not get to squash that mosquito in time to prevent the Plasmodia to be injected into your bloodstream. Additionally, a jet-lagged individual may experience a peak of body temperature at night. Mosquitoes, among else, home in on the warmth of their victims. Thus, jet-lagged individuals may be warmer than the surrounding locals at midnight and thus more attractive to mosquitoes at that time.
On the other hand individuals visiting endemic areas from different time zones, particularly during the first couple of days are under the state of jet lag, and their peak protective daily behavioural itch sensitivity lies out of phase with the biting peak of female mosquitoes. Therefore, such individuals are at a greater risk for sustaining malaria compared to the residents. Thus from chronobiological perspective one is of the opinion that a person can be protected against malaria by appropriately adjusting circadian clocks regulating itch sensitivity to the periodic environment. We hope that recent developments in circadian biology will help us predict extent of adjustments necessary in a new environment, which can then be of paramount importance for the protection of jet-lagged travelers to endemic regions against malaria. Some protection against malaria in the chronotherapeutic procedures such as melatonin administration, light therapy, scheduled physical exercise, maximum exposure to new environment during vector free times, social interactions, and appropriate food habits, are a few recommended preventive measures for travelers visiting a malaria endemic areas, in addition to malarial antibiotic prophylaxis.Sounds like good advice, although the administration of melatonin is always an iffy question. However, this hypothesis got my mind all twirling and I came up with some hypotheses of my own. However, it is important to distinguish between different kinds of hypotheses regarding a putative link between jet-lag and malaria. They suggest that jet-lag may:
1) affect the rate/ease of infection with malaria,
2) affect the symptoms of malaria in an infected individual,
3) affect the ability of the body to fight off the infection,
4) affect the effectiveness of treatments, and
5) affect the likelihood that the infected individual will spread the disease to others.
The Kumar/Sharma hypothesis is clearly of the #1 type. I will look more at other types of hypotheses - those that apply to already infected individuals. For that, let's first go quickly through the basic biology of malaria.
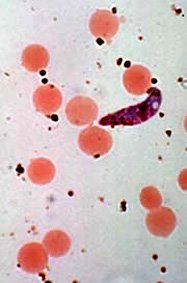
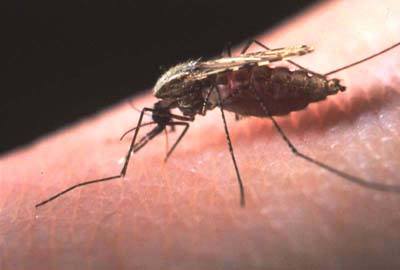
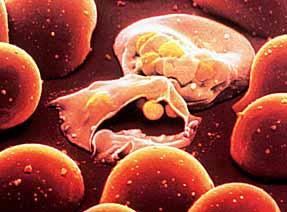
All the plasmodia burst out of red blood cells simultaneously. Enormous number of plasmodia suddenly released into the blood overwhelms the immune system of the host, allowing the plasmodia to survive unscathed for quite a long time. This time is sufficient for them to invade blood vessels in the skin where, if they are lucky, a mosquito will bite and the plasmodia can invade the mosquito again and search for the next host.
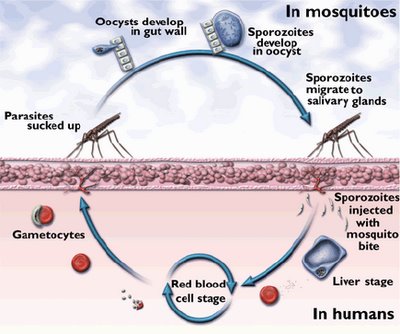
Obviously, from the perspective of a Plasmodium, timing is crucial. First, it is important to errupt in synchrony. Yet, hidden inside red blood cells, plasmodia cannot communicate with each other. Second, it is important to time the eruption in such a way as to maximize the probabilty that some of the gametocytes will be picked up by mosquitoes. Thus, it is important for the eruption to occur at the time of day when mosquitoes are most actively foraging for blood.
How do the Plasmodia solve the problem of timing? This is where circadian biology comes in [3,4,5]. Plasmodia residing inside red blood cells use the time-clues generated by the host. More specifically, they key onto the nightly release of melatonin by the pineal gland. Melatonin is practically undetectable in the blood during the day and the concentrations rise steeply in the evening remaining high for the duration of the night (exact patterns differ between vertebrate species), then dropping again at dawn.
Plasmodia have melatonin receptors [3]. Interestingly, unlike melatonin receptors in vertebrates which are nuclear receptors, the receptors in Plasmodia are membrane receptors. Membrane receptors are much faster than nuclear receptors which is important when a biological event has to be timed with precision. However, the plasmodia do not destroy the red blood cell immediately after receiving the melatonin signal - that would be too early in the evening for the timing to be adaptive, as the mosquitoes are still too busy looking for mates and mating at that time. Instead, the plasmodia use their own circadian clocks to measure the exact timing of eruption. In a way, it appears that the host melatonin signal entrains (synchronizes) the clocks in plasmodia, and then the Plasmodium clock determines the phase (exact timing) for the eruption out of red blood cells.
Different species of Anopheles and even geographically distinct populations of the same species have different times of peak foraging (biting) activity. In each geographical region, the local population (or species) of Plasmodium evolved the timing of eruption to match that of the local mosquitoes.
Let's now introduce another player. Apart from the parasite (Plasmodium), the host (a vertebrate, e.g., a human), and the vector (mosquito), one should also consider the predator - insectivorous bats that hunt for mosquitoes. The way that the malaria literature tends to think about timing can schematically be presented like this:
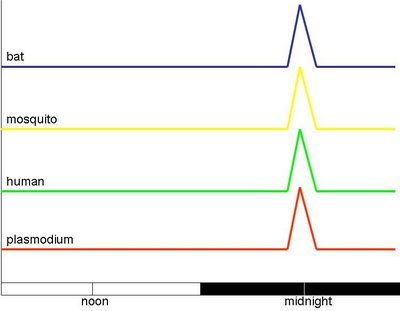
On the other hand, it is maladaptive for mosquitoes and plasmodia, and adaptive for humans and bats, if the peak hunting time for bats coincides with the peak foraging time of mosquitoes. More these two events are in sync, more mosquitoes will get eaten, thus less plasmodia will get into a new host and less humans will get infected.
The dynamics of the timing relationship between the four species can be described as an Evolutionary Arms-Race Around The Circadian Clock. While some of the players will try to maximize their fitness by achieving synchrony, the other players maximize their fitness by avoiding synchrony with each other. This can be depicted, for bats and mosquitoes, like this:
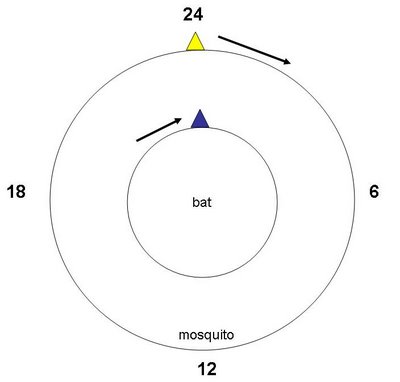
Plasmodium species are pretty host-specific. Species that thrive inside humans, may not thrive or even survive inside the bodies of other animals and vice versa. So, the parasite is pretty loyal to its host. It is also completely dependent on Anopheles - it will most likely not survive inside a different kind of mosquito.
The same mosquito that usually bites a human will happily take a blood meal from another animal. This is actually used as one of the prevention techniques: a village is surrounded by fields full of cattle, sheep, goats, horses, donkeys or camels. The mosquitoes coming out of the woods at night encounter these animals first and get satiated with blood before they ever encounter humans. The animals themselves do not get sick.
Bats are unlikely, in my opinion, to be specialized on Anopheles as their only prey. If there are no mosquitoes around, they will happily hunt other insects (and the tropical regions where malaria is common are swarming with many species of insects!). I think that involvement of bats in the arms-race is the weakest aspect of the hypothesis. Here are four basic types of bat hunting activity that are theoretically possible:
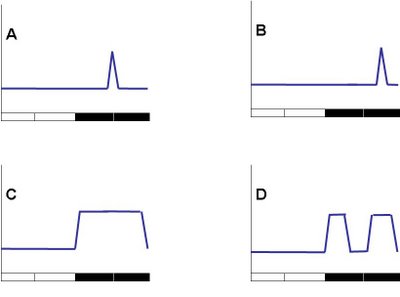
What is the real story? I don't know. Obviously, it is possible to monitor patterns of bat activity [6,7], yet it still needs to be done in regions in which malaria is common. Some of the bats studied in the USA follow predominantly pattern C from the figure above, and it is not too far-fetched to hypothesize that all bats everywhere have similar patterns:
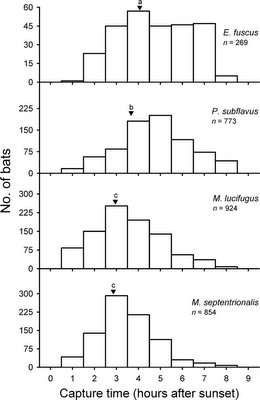
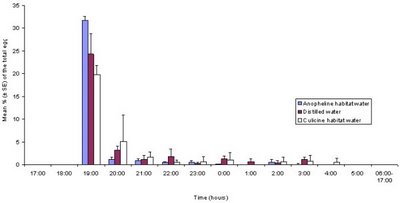
Will jet-lag affect the way our body copes with the infection? In a jet-lagged human, there is no clear and sharp rhythm of melatonin release. Some amounts of melatonin are synthetized and secreted at all times of day. This means that the Plasmodium has lost its temporal anchor - there is no signal to use for determination of timing for eruption out of red blood cells. Thus, the gametocytes will errupt at random times - one cell now, another in an hour, another tomorrow. There is no safety in numbers any more - the human immune system is now perfectly capable of dealing with all the plasmodia in the circulation. Of course, the immune system itself may be somewhat compromised in a jet-lagged person.
Will jet-lag affect the way malaria presents its symptoms? The asynchronous eruption of plasmodia also means that there will be no abrupt onset of high fever at midnight. Instead, one may expect a continous low-grade fever. Nightly episodes of high fever are an important symptom of malaria. Will a physician with a patient who exhibits continuous low-grade fever ever suspect malaria? Especially a physician in a country in which there is no malaria and the patient has returned home from the tropical travels and is jet-lagged from a return trip.
Will jet-lag affect the effectiveness of drug treatments? I don't know the details of the way anti-malarial drugs work, so make sure you tell me if I get this all wrong. If the number of plasmodia in the circulation at any time is relatively small, and if the enzymatic destruction of the drug by liver is operating at a constant low rate (instead of with a circadian rhythm of its own), then being jet-lagged should enhance the effectiveness of the drugs, or even allow for the dose to be lowered.
Will jet-lag affect the ability of the patient to be a source of transmittion of the disease to others? With plasmodia erupting at all times of day and with most plasmodia being destroyed by the immune system throughout the day, it is much less likely that any will be present in the skin capillaries at just the right time - at midnight. Also, without a high fever coupled with sweating, the patient is less attractive to the mosquitoes than a malarial patient in the neighboring house who is local and not jet-lagged. Thus, the likelihood of plasmodia being picked up by mosquitoes is even smaller.
To summarize: according to the Kumar/Sharma hypothesis, being jet-lagged increases the chances for contracting malaria. On the other hand, if you already have the disease, it may be good for you to get jet-lagged! As long as you tell your physician that malaria is a serious option so the symptoms are not misinterpreted, you should be better off jet-lagged, allowing your body to fight the disease one plasmodium at a time.
Finally, as a matter of public health policy, how does one get the whole population of malarial patients in one country jet-lagged so as to reduce the transmission rates? Should hospitals induce jet-lag in malaria patients by shifting light-cycles or administering melatonin? How do the pros and cons of such treatment balance? Ah, so many hypotheses, so little data! I hope someone studies this in the future.
One last thing - notice that much of the work described above was performed by researchers outside of USA. Apart from a little bit of cellular physiology, most of the information comes from ecological field-work, and ALL of it is inspired by and informed by evolutionary theory. Not a single gel was run.
Now, I am not dissing molecular biology. Malaria is the only complex parasitic disease in which all players (plasmodium, mosquito and human) have their complete genomes sequenced, and much will be gleaned from such data in terms of designing better anti-malarial drugs, etc. But, as the above research shows, Big (molecular) Biology is not neccessary for findings that have a potential to seriously affect the infection and transmission rates of the disease.
[1] Jet lag and enhanced susceptibility to malaria, C. Jairaj Kumar and Vijay Kumar Sharma, Medical Hypotheses (2006) 66, 671–685
[2] Fooling Anopheles: Scientists Aim to Wipe Out Malaria by Outsmarting a Mosquito's Sense of Smell
[3] Calcium-dependent modulation by melatonin of the circadian rhythm in malarial parasites, Carlos T. Hotta, Marcos L. Gazarini, Flávio H. Beraldo, Fernando P. Varotti, Cristiane Lopes, Regina P. Markus, Tullio Pozzan and Célia R. S. Garcia, NATURE CELL BIOLOGY , VOL 2, JULY 2000, p.468
[4] Melatonin and N-acetyl-serotonin cross the red blood cell membrane and evoke calcium mobilization in malarial parasites, C.T. Hotta, R.P. Markus and C.R.S. Garcia, Braz J Med Biol Res 36(11) 2003
[5] Tertian and Quartan Fevers: Temporal Regulation in Malarial Infection, Célia R. S. Garcia, Regina P. Markus, and Luciana Madeira, JOURNAL OF BIOLOGICAL RHYTHMS, Vol. 16 No. 5, October 2001 436-443
[6] Sampling bats for six or twelve hours in each night? Esberard CEL, Bergallo HG, REVISTA BRASILEIRA DE ZOOLOGIA 22 (4): 1095-1098 DEC 2005
[7] Nightly, seasonal, and yearly patterns of bat activity at night roosts in the Central Appalachians, Agosta SJ, Morton D, Marsh BD, Kuhn KM, JOURNAL OF MAMMALOGY 86 (6): 1210-1219 DEC 2005
[8] Daily oviposition patterns of the African malaria mosquito Anopheles gambiae Giles (Diptera: Culicidae) on different types of aqueous substrates, Leunita A Sumba, Kenneth Okoth, Arop L Deng, John Githure, Bart GJ Knols, John C Beier and Ahmed Hassanali, Journal of Circadian Rhythms 2004, 2:6
Saturday, March 18, 2006
Circadian Rhythms in Human Mating
I remember from an old review that John Palmer did a study on the diurnal pattern of copulation in humans some years ago. You can see the abstract here.
Now, Roberto Reffinetti repeated the study and published it in the online open-source Journal of Circadian Rhythms here.
The two studies agree: The peak copulatory activity in people living in a modern society is around midnight (or, really, around bedtime) with a smaller secondary peak in the morning around wake-time.
Dig through the papers yourself for additional data on workday-weekend differences and the temporal patterns of the female orgasm.
Now, Roberto Reffinetti repeated the study and published it in the online open-source Journal of Circadian Rhythms here.

Dig through the papers yourself for additional data on workday-weekend differences and the temporal patterns of the female orgasm.
Tuesday, March 14, 2006
Sleep societies
Don't worry too much about the source of this information - the list of sleep societies is correct.
Expecting a baby any day now?
If so, you may want to track the infant's sleep patterns, feeding, diaper-changes, etc. Now made easy with Trixie Tracker. The patterns will make your life more predictable, will let you notice when something goes wrong before it becomes too apparent, and will make childrearing even more fun.
Of course, you can use the software for tracking an adult, yourself included. You can replace baby formula with beer and track your drinking habit. It is quite flexible. Go take a look.
[Note: I just like the software - I do not get anything from advertising it here, and I have only briefly met the author at a blogging conference about a year ago]
Of course, you can use the software for tracking an adult, yourself included. You can replace baby formula with beer and track your drinking habit. It is quite flexible. Go take a look.
[Note: I just like the software - I do not get anything from advertising it here, and I have only briefly met the author at a blogging conference about a year ago]
Monday, March 13, 2006
What is a 'natural' sleep pattern?
I have mentioned this in my very first post here: in a natural state, humans do not sleep a long consecutive bout throughout the night. The natural condition is bimodal - two bouts of sleep interrupted by a short episode of waking in the middle of the night.
In today's New York Times, there is an article about this:
Sleep Disorder? Wake Up and Smell the Savanna by RICHARD A. FRIEDMAN, M.D.:
I have nothing to add, except I can also give you an image I dug up - the original data from Wehr's experiment. See how the sleep is bimodal during the long winter nights and gets compressed during simulated summer:
In today's New York Times, there is an article about this:
Sleep Disorder? Wake Up and Smell the Savanna by RICHARD A. FRIEDMAN, M.D.:
------------snip-----------
Many patients tell me they have a sleep problem because they wake up in the middle of the night for a time, typically 45 minutes to an hour, but fall uneventfully back to sleep. Curiously, there seems to be no consequence to this "problem." They are unaffected during the day and have plenty of energy and concentration to go about their lives.
------------snip-----------
The problem, it seems, is not so much with their sleep as it is with a common and mistaken notion about what constitutes a normal night's sleep.
It's a question that Dr. Thomas Wehr at the National Institute of Mental Health asked himself in the early 1990's. He conducted a landmark experiment in which he placed a group of normal volunteers in 14-hour dark periods each day for a month. He let the subjects sleep as much and as long as they wanted during the experiment.
------------snip-----------
By the fourth week, the subjects slept an average of eight hours a night — but not consecutively. Instead, sleep seemed to be concentrated in two blocks. First, subjects tended to lie awake for one to two hours and then fall quickly asleep. Dr. Wehr found that the abrupt onset of sleep was linked to a spike in the hormone melatonin. Melatonin secretion by the brain's pineal gland is switched on by darkness.
After an average of three to five hours of solid sleep, the subjects would awaken and spend an hour or two of peaceful wakefulness before a second three- to five-hour sleep period. Such bimodal sleep has been observed in many other animals and also in humans who live in pre-industrial societies lacking artificial light.
Carol Worthman, an anthropologist at Emory University in Atlanta, has studied the sleep patterns of non-Western populations. From the !Kung hunter-gatherers in Africa to the Swat Pathan herders in Pakistan, Dr. Worthman documented a pattern of communal sleep in which individuals drifted in and out of sleep throughout the night.
She speculates that there may even be an evolutionary advantage to interrupted sleep. "When we lived in open exposed savanna, being solidly asleep leaves us vulnerable to predators."
With artificial light, modern humans have essentially managed to extend their daytime activities late into the night, when all other sensible creatures are busy sleeping.
As a result, we have compressed our natural sleep into artificially short nighttimes, but not all people are so easily tamed by artificial light. Some people, who may just have very strong circadian rhythms, still have this primitive bimodal sleep that they confuse with a sleep disorder.
Add these people to the rest of us who, under the pressures of modern life, often have some trouble falling or staying asleep and there is a large captive audience for drug companies.
Thanks in large part to the meteoric rise in direct-to-consumer advertising, medications like Ambien and Lunesta have become household names and seductive panaceas that millions find hard to resist — even though a majority have no serious sleep problem to repair. If it's any consolation to those of you who are awake in the middle of the night for an hour or so, reading or watching television, you may simply be the most natural sleepers.
I have nothing to add, except I can also give you an image I dug up - the original data from Wehr's experiment. See how the sleep is bimodal during the long winter nights and gets compressed during simulated summer:

ClockNews
Kinder, Gentler Chemotherapy:
Don't blame the other woman, she says:
Forest Hill High to test 9 a.m. school-day start:
"Every drug has an optimal time when it is least toxic and most effective," says Dr. Block. "For cancer treatment, this is determined by several factors, including the biological uniqueness of the particular drug being given, the time when the specific type of cancer cells divide the most, when the normal healthy cells of the patient generally divide the least, the patient’s circadian clock and individual rest-activity cycles, and even the time zone the person resides in."
"We have found that often patients receiving their chemotherapy this way reduce what would have been recurring side effects of nausea, vomiting, diarrhea, and fatigue," explained Dr. Block cofounder and Medical/Scientific Director of the Block Center for Integrative Cancer Care, and Director of Integrative Medicine Program at the University of Illinois College of Medicine at Chicago. This is important because the debilitation caused by chemo can cause patients to reduce or even stop treatments that could otherwise help them win their battle with cancer." In fact, current research shows that up to 1/3 of chemotherapy patients abandon treatments prematurely due to the side effects.
Don't blame the other woman, she says:
Couples often don't consider that their libidos may be on incompatible circadian rhythms, but it's not unusual.
Forest Hill High to test 9 a.m. school-day start:
In fact, recent research finds that teenagers are not unlike narcoleptics at that time in the morning. In lab tests, teenagers fell asleep quickly and into the deep REM sleep state, said Mary Carskadon, the Brown University professor who conducted the study.
Carskadon said teenagers' circadian rhythms — essentially, their internal clocks — along with distractions such as the Internet make it difficult for them to go to bed before 10:30 or 11 p.m. That means they usually don't get enough sleep before early classes.
"It's a double whammy," Carskadon said. "They're not getting enough sleep to recharge their brains, and we're asking their brains to be on duty at the wrong time."
Wednesday, March 08, 2006
Clocks in Bacteria I: Synechococcus elongatus
As I stated in the introductory post on this topic, it was thought for a long time that prokaryotes were incapable of generating circadian rhythms. When it was discovered, in 1994 [1], that one group of prokaryotes, the cyanobacteria, possess a circadian clock, the news was greeted with great excitement. This was the first definitive demonstration of a circadian clock in a bacterium (I intend to revisit the E.coli saga in a later post).
All three hypotheses for the origin of the circadian clock suppose that it first evolved in an aquatic, unicellular organism. While protists fit the bill quite nicely, having a bacterium with a circadian clock pushed the origin of the clock further back into the past. This made the researchers happy as it supported the notion that the clock was a universal property of life, as well as that it evolved only once in the history of Life on Earth. This also suggested that clocks in all organisms use same or similar intercellular mechanisms for generation of circadian rhythms.
At the time that clocks were discovered in cyanobacteria, only two circadian genes were characterized: period in fruitflies and frequency in Neurospora crassa. The second fly gene, timeless, was diescovered the following year, and the first mammalian gene Clock and the first plant gene Toc were discovered some years later. Thus, at the time, it was still plausible that all of life used the same mechanism for the circadian clock, just as all of life uses ATP for energy storage and DNA for information storage.
However, studying genetics in bacteria is a much quicker and easier task than in the large multicellular eukaryotes. Very soon, the cyanobacterial clock genes were discovered and it turned out that they had no resemblance to fly or mold genes. KaiA, KaiB and KaiC (as they were discovered in Japan, they were named "kaiten", which implies a cycle of events reminiscent of the turning of the heavens) have no homologies with any of the clock genes found in any other group of organisms and the internal logic of the bacterial clock is different from that in plants, fungi and animals, i.e., it is not a typical transcription-translation feedback loop.
The clock in cyanobacteria is better thought of as a relay switch. It turns about 2/3 of the genome on in the morning (and off in the evening) and turns on the remaining 1/3 of the genome at dusk (and off at dawn). Recent findings about bacterial, plant, protist, fungal and animal clocks suggests as many as five separate events of the origin of a circadian clock on Earth - one for each major group of organisms.
Mutations and deletions [1,2 5,6] of either one of the three Kai genes affect the circadian phenotype, either by altering the inherent period of the freerunning rhythm, or by abolishing rhythmicity altogether. Interestingly, Synechococcus cells appear to have a "memory" of the circadian phase in which they find themselves and this memory gets transmitted from parental to daughter cells during cell division. Actually, under certain conditions, cell division is a much more rapid process than a circadian cycle. In other words, Synechococcus may undergo several cell divisions over a period of a single day, yet the colony as a whole keeps its circadian rhythms running all along [2,3].
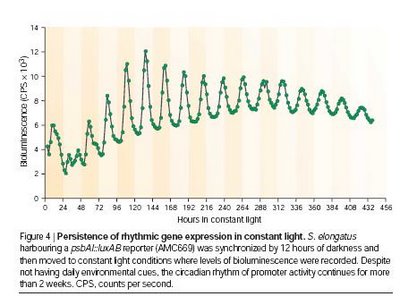
Next time, I will focus on the contributions of cyanobacteria to the understanding of the origin, evolution and adaptive function of circadian clocks.
References and further reading:
[1] Circadian clock mutants of cyanobacteria by Kondo T, Tsinoremas NF, Golden SS, Johnson CH, Kutsuna S, Ishiura M., Science.266(5188):1233-6 (1994, Nov 18)
[2] Circadian clocks in prokaryotes by Carl Hirschie Johnson, Susan S. Golden, Masahiro Ishiura & Takao Kondo, Molecular Microbiology, Volume 21 Page 5 (July 1996).
[3] Circadian Rhythms in Rapidly Dividing Cyanobacteria by Takao Kondo, Tetsuya Mori, Nadya V. Lebedeva, Setsuyuki Aoki, Masahiro Ishiura and Susan S. Golden, Science, Vol. 275. no. 5297, pp. 224 - 227 (10 January 1997)
[4] Independence of Circadian Timing from Cell Division in Cyanobacteria by Tetsuya Mori and Carl Hirschie Johnson, Journal of Bacteriology, p. 2439-2444, Vol. 183, No. 8 (April 2001)
[5] CYANOBACTERIAL CIRCADIAN CLOCKS — TIMING IS EVERYTHING by Susan S. Golden & Shannon R. Canales, Nature Reviews Microbiology 1, 191-199 (2003)
[6] Circadian rhythms: as time glows by in bacteria by Johnson CH, Nature 430, 23-24 (2004)
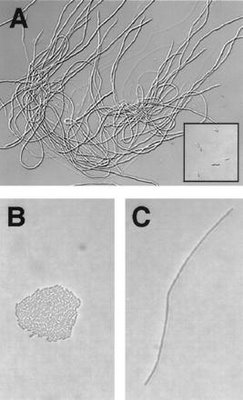
At the time that clocks were discovered in cyanobacteria, only two circadian genes were characterized: period in fruitflies and frequency in Neurospora crassa. The second fly gene, timeless, was diescovered the following year, and the first mammalian gene Clock and the first plant gene Toc were discovered some years later. Thus, at the time, it was still plausible that all of life used the same mechanism for the circadian clock, just as all of life uses ATP for energy storage and DNA for information storage.
However, studying genetics in bacteria is a much quicker and easier task than in the large multicellular eukaryotes. Very soon, the cyanobacterial clock genes were discovered and it turned out that they had no resemblance to fly or mold genes. KaiA, KaiB and KaiC (as they were discovered in Japan, they were named "kaiten", which implies a cycle of events reminiscent of the turning of the heavens) have no homologies with any of the clock genes found in any other group of organisms and the internal logic of the bacterial clock is different from that in plants, fungi and animals, i.e., it is not a typical transcription-translation feedback loop.
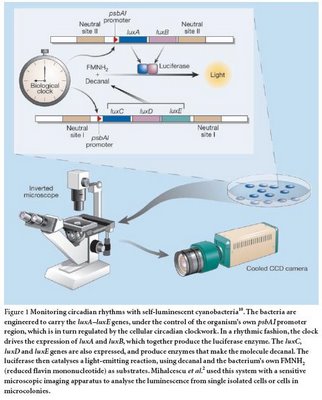
Mutations and deletions [1,2 5,6] of either one of the three Kai genes affect the circadian phenotype, either by altering the inherent period of the freerunning rhythm, or by abolishing rhythmicity altogether. Interestingly, Synechococcus cells appear to have a "memory" of the circadian phase in which they find themselves and this memory gets transmitted from parental to daughter cells during cell division. Actually, under certain conditions, cell division is a much more rapid process than a circadian cycle. In other words, Synechococcus may undergo several cell divisions over a period of a single day, yet the colony as a whole keeps its circadian rhythms running all along [2,3].
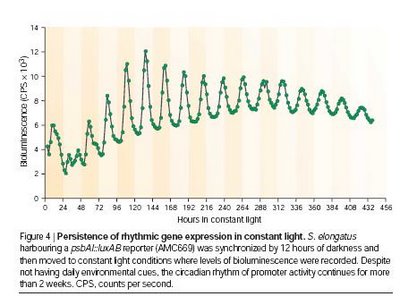
Next time, I will focus on the contributions of cyanobacteria to the understanding of the origin, evolution and adaptive function of circadian clocks.
References and further reading:
[1] Circadian clock mutants of cyanobacteria by Kondo T, Tsinoremas NF, Golden SS, Johnson CH, Kutsuna S, Ishiura M., Science.266(5188):1233-6 (1994, Nov 18)
[2] Circadian clocks in prokaryotes by Carl Hirschie Johnson, Susan S. Golden, Masahiro Ishiura & Takao Kondo, Molecular Microbiology, Volume 21 Page 5 (July 1996).
[3] Circadian Rhythms in Rapidly Dividing Cyanobacteria by Takao Kondo, Tetsuya Mori, Nadya V. Lebedeva, Setsuyuki Aoki, Masahiro Ishiura and Susan S. Golden, Science, Vol. 275. no. 5297, pp. 224 - 227 (10 January 1997)
[4] Independence of Circadian Timing from Cell Division in Cyanobacteria by Tetsuya Mori and Carl Hirschie Johnson, Journal of Bacteriology, p. 2439-2444, Vol. 183, No. 8 (April 2001)
[5] CYANOBACTERIAL CIRCADIAN CLOCKS — TIMING IS EVERYTHING by Susan S. Golden & Shannon R. Canales, Nature Reviews Microbiology 1, 191-199 (2003)
[6] Circadian rhythms: as time glows by in bacteria by Johnson CH, Nature 430, 23-24 (2004)
Monday, March 06, 2006
So, is Cryptochrome a clock gene in fruitflies, too?
From the press release earlier today:
NYU, Univ. Of London Study Offers Additional Evidence Mammals & Fruit Flies Share Make-Up On Function of Biological Clocks
The paper itself is not available online yet. If there is something in it that I feel needs to be commented on, I will post it later.
NYU, Univ. Of London Study Offers Additional Evidence Mammals & Fruit Flies Share Make-Up On Function of Biological Clocks
A study by researchers at New York University and the University of London offers additional evidence that mammals and fruit flies share a common genetic makeup that determines the function of their internal biological clocks. The study appears in the latest issue of Current Biology.
The research team consisted of post-doctoral researcher Ben Collins, Esteban Mazzoni, a graduate student, and Assistant Professor Justin Blau of NYU’s Department of Biology and Professor Ralf Stanewsky of the University of London.
Drosophila fruit flies are commonly used for research on biological, or circadian, clocks because of the relative ease of finding mutants with non-24-hour rhythms and then identifying the genes underlying the altered behavior. These studies in fruit flies have allowed the identification of similar “clock genes” in mammals, which function in a similar manner in mammals as they do in a fly’s clock. However, prior to this study, biologists had concluded that the role of one protein-Cryptochrome (Cry)-was quite different between flies and mammals. In fruit flies, Cry is a circadian photoreceptor, which helps light reset the biological clock with changing seasons, or in jet lag-style experiments (in which light is manipulated to mimic the experience of traveling over multiple time zones) in the lab. In mammals, however, Cry assists in the 24-hour rhythmic expression of clock genes and has nothing to do with re-setting the clock.
The researchers sought to determine additional roles for Cry in fruit flies by testing the rhythmic expression of clock genes in flies with either a mutant version of Cry, or with Cry produced at artificially high levels. In both cases, they found that the clock had stopped - with high levels of clock gene expression when Cry was mutated, and low levels when Cry was over-produced. These results indicated that Cry normally inhibits clock gene expression in many clock cells - just as it does in the mammalian clock.
“In addition to finding a new function for Cryptochrome, the results reinforce that notion that fruit flies provide an excellent model for understanding the human biological clock that drives sleep/wake cycles and many other processes that contribute to our overall health,” said Blau.
The paper itself is not available online yet. If there is something in it that I feel needs to be commented on, I will post it later.
Sleep or Excercise?
What is more important: an additional hour of sleep or an hour of exercise? Dr. Michael Breus answers.
Friday, March 03, 2006
Sleep monitoring under realistic conditions
A new awakening for sleep research
Whether you are having problems to stay awake or falling asleep, sleep science has yet offered little help, due to costly and complicated diagnostics and treatments. Researchers aim to drastically change this with a new way of measuring sleep.
The IST project SENSATION is an ambitious project of 46 partners from 20 different countries, addressing sensing of physiological parameters, core computation, medical and industrial research. The aim is to take sleep research to a whole new level by developing a multipurpose sensing platform consisting of 17 micro sensors and two nano sensors, connected through a local area network.
"The sensors will allow you to sleep at home on, for instance, a mattress with sensors instead of going to a hospital which is much more comfortable and the test becomes more precise," explains Dr Evangelos Bekiaris, project coordinator. Today, you will have to go to a hospital sleep lab for 1-2 nights and have your sleep measured to evaluate your sleep. "These tests are costly and since monitoring sleep cannot be done in your home environment they are not as reliable," says Bekiaris.
The sensors will be integrated into a wide range of materials such as bed and pillow textiles, wrist straps, seat linings and the frames of glasses. Wirelessly integrated through a computer network they will measure your brain activity, heart rate, eye and muscle movements during your waking and sleeping hours. The data will be collected in a body area network, wirelessly transferred to a local area network and then sent to the hospital for analysis, Bekiaris explains. The sensors can also be used for safe monitoring and early warning of people while driving or supervising a critical task, like the operators of nuclear power stations or air traffic controllers, before they fall asleep and cause an accident.
The project is now halfway through its funding period and is already showing a lot of tangible results. The first stage of the project involved data collection, setting up of databases and formulating recommendations for the development of sensors.
"We have developed something which is truly unique, with two extensive databases of sleep data, one with data of normal sleep with 350 participants and another one with sleep data of 400 people monitored while working or driving, crossing the stage between vigilant to sleep," says Bekiaris. "About half of these people were tested in a driving simulator but the other half was tested driving on highways with double command cars. The tests show a significant difference in the persons reactions, as the persons in the simulator were more calm, knowing they were part of a test, whereas the people on the highways were really fighting sleep." It shows that when monitoring people in their daily lives there are significant differences in result, he continues.
The project, spanning such a vast research area, has the potential for a strong impact. According to statistics 25 per cent of the traffic accidents in the UK, or 40 per cent in the US, is related to driver fatigue. The project estimates that the SENSATION sleep platform with its sensors could reduce serious road accidents by 30 per cent and industrial accidents by over 15 per cent.
Although one of the main areas is to develop sensors to measure the waking state of a person, both the collected data from the project and the final results can prove to be useful for further research. "Take falling asleep at workplaces as an example," he says. "Today you have techniques that might alert you as you fall asleep, but what we want is a system that will alert the person already before this happens. In some work environments or while driving it might already be too late if you have started dozing off."
The applications are vast. You could monitor aircraft pilots to make sure they are awake. It could be used to monitor babies and prevent cot death, or in sleep management, to evaluate your own sleep and from this plan shift work according to people's biorhythms, Bekiaris explains.
"We currently have nine sensors ready and plan to present the first sensors and prototypes at the International conference on 'Monitoring sleep and sleepiness - from physiology to new sensors' which will be held in Switzerland 29-30 May 2006," says Bekiaris.
Real-life tests of the prototypes and the entire sleep platform are planned to start by the beginning of next year.
Wednesday, March 01, 2006
Circadian Leaf Movements in Plants
The very first circadian rhythm ever observed was a rhythm of opening and closing of leaves of a mimosa plant. That was a couple of centuries ago (1729, to be exact), reported by astronomer de Mairan. Since then, many researchers, from Charles Darwin, through 19th century botanists Zinn and Hoffer, to 20th century chronobiologist Erwin Bunning, studied this rhythm.
Now, you can see three examples of such movements (check the three plant species on the left sidebar there) on a website that provides the movies. Cool, isn't it?
(hat-tip: Discovering biology in a digital world)
Now, you can see three examples of such movements (check the three plant species on the left sidebar there) on a website that provides the movies. Cool, isn't it?
(hat-tip: Discovering biology in a digital world)